- Departments
- Microsensor Group
- Dirk de Beer
- Research Projects
Research Projects
Transport and mineralization processes in intertidal zones
Beaches and plates (with Marit van Erk)
Kelp can accumulate in enormous amounts on beaches. This sudden input of organic material leads to a different degradation scenario as in normal early diagenesis. Marit has worked with Dimitri Meyer (Univ. Vienna) on a combined functional and biodiversity study. The intensity of the degradation rates allowed to detect all steps: hydrolysis, fermentation, aerobic and anaerobic respiration. Normally, degradation follows a series of thightly coupled steps, where products of anaerobic steps are oxidized by oxidized intermediates and eventually by oxygen. Whereas normally all stays internal in the sediments, from kelp deposits large amounts of reduced compounds escape, both organic and inorganic and partially toxic. For example, the sea adjacent to the kelp deposits become sulfidic, and massive amounts of sulfuroxidising mats develop quickly. This aerobic community was surprisingly diverse, and contained known anaerobes that adopted an aerobic lifestyle and had lost their denitrifying genes. The varying O2 levels induced by tides lead to significant CO development by reaction with the humic acids in the kelp deposits. CO is converted anaerobically via H2 by sulfate reduction and also is released to kill passing animals and tourists (www.bbc.co.uk/news/world-europe-14324094). The very sudden and strong increase in degradation processes upon kelp additions to sediment indicate the community is tuned to kelp and hungrily waiting for it. To demonstrate the specificity of the community, Marit is now studying the hydrolysis of kelp to specific sugars and the responses to different carbohydrates.
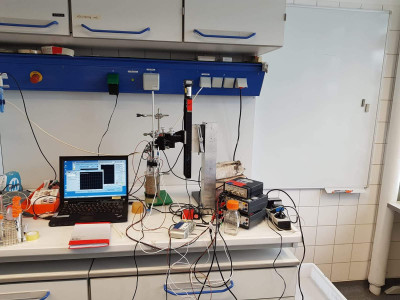
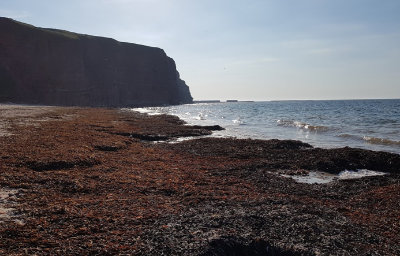
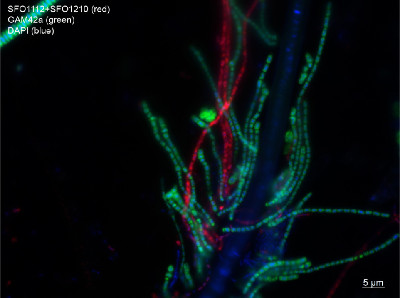
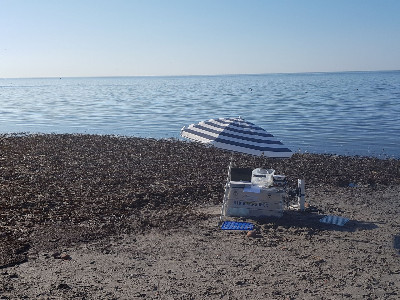
Sulfur bacteria
With Charles Schutte, Verena Salman-Carvalho, Barbara MacGregor, Marit van Erk
The sulfide oxidising Beggiatoa (nowadays called Beggiatoacea) are among the largest bacteria found. They form multicellular filaments with a length of cms and a thickness of upto half a mm. Most of their cell volume is a vacuole filled with upto 500 mM nitrate. Beggiatoa form large white mats on sulfidic and organic rich sediments. The filaments are highly motile, and migrate between the sediment surface and the sulfidic zone deep in the sediments. They survive the anoxia using the stored nitrate, which allows them to stay for a month in the anoxic zones.
We investigate how they orient themselves in the sediments (if you move you must navigate, e.g. to find the surface back), their phylogeny, how they oxidise sulfide, and how they grow.
An old debate is if Beggiatoa oxidises sulfide via denitrification or via dissimilatory nitrate reduction to ammonium (DNRA). Important as ammonium remains as nutrient in the ecosystem, nitrogen not. It appears they can do both.
Beggiatoa is in deep-sea vents the main primary producer of biomass. CO2 fixation is performed by RuBisCo. We showed that their growth rate is proportional to the CO2 partial pressure. That is much higher at 2 mm depth than at the surface. Thus migrating downwards supplies Beggiatoa with sulfide and CO2.
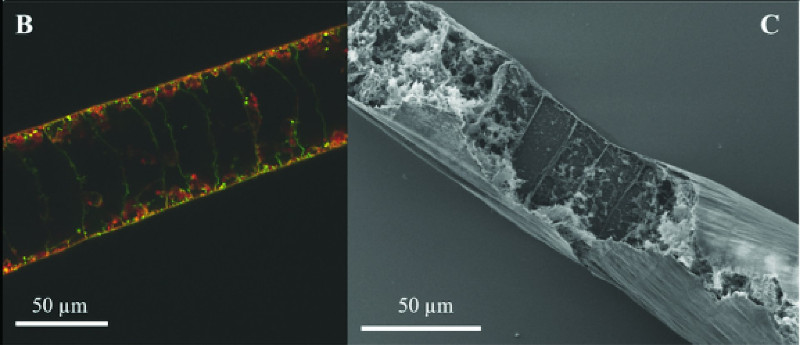
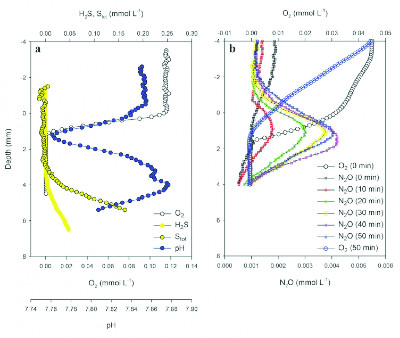
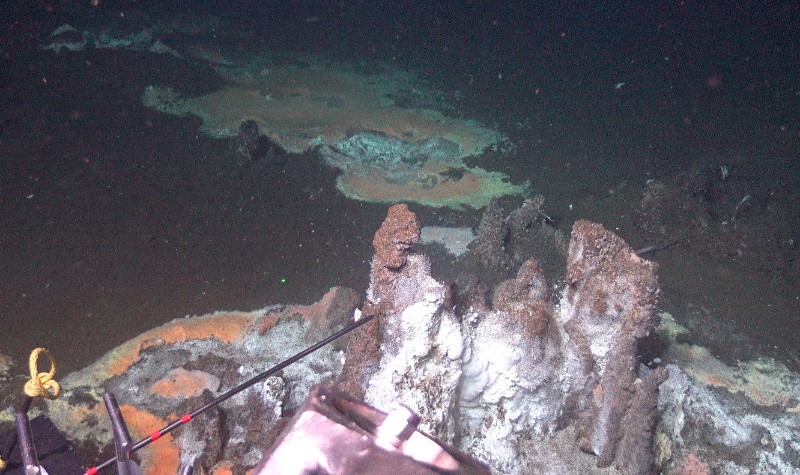
Sulfur blobs
On a smoker (hot sulfidic vent) in the Guaymas basin we observed conspicious white gels. They consisted of fibrous material and had a highly diverse microbial community, including Arcobacter. The fibrous material appeared to be sulfur. Arcobacter is a strictly aerobic sulfide oxidiser. On board of the ship they decomposed within a day, physiological tests were done directly after sampling with microsensors.
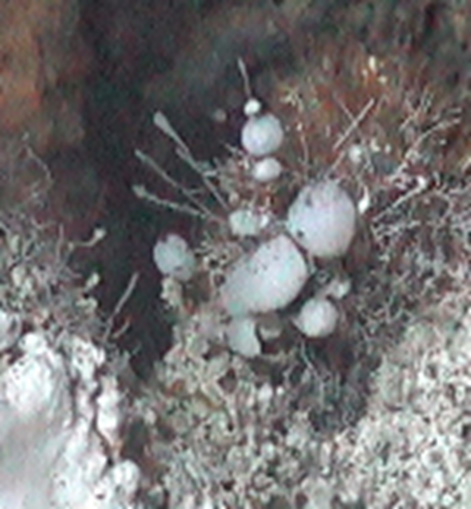
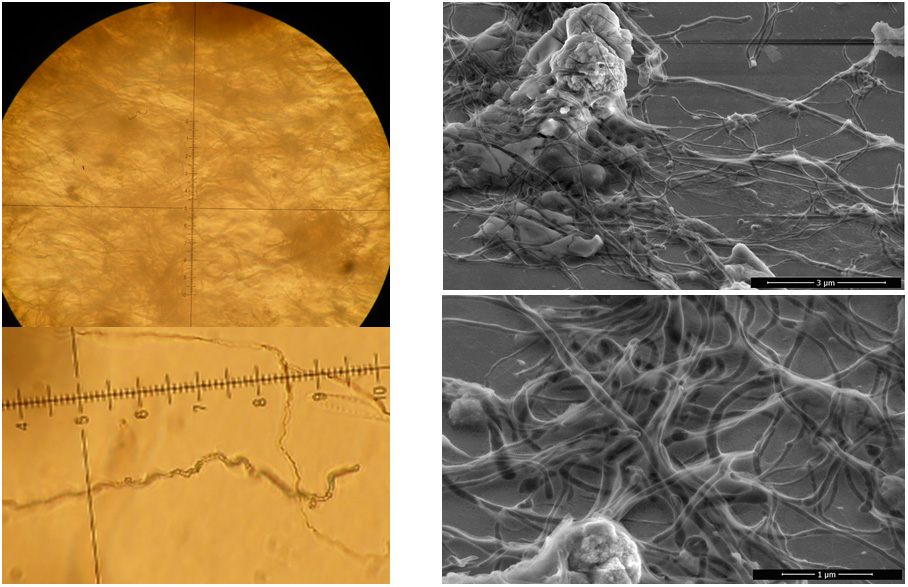
Phototrophic microbial mats
With Dimitri Meyer (University Vienna), Arjun Chennu (https://www.mpi-bremen.de/en/Arjun-Chennu.page), Andreas Greve ([Bitte aktivieren Sie Javascript])
Microbial mats inhabited the world before the great oxidation event (GOE), and formed the oldest known fossils. Part of these mats were structured by Cyanobacteria, the microbes that can perform anoxygenic photosynthesis and 'invented' oxygenic photosynthesis. After the GOE, complex life developed that destroyed the mats by grazing. Nowadays, mats are only found at places where no higher lifeforms can survive: hot-, hypersaline or anoxic habitats. Anoxic illuminated habitats are very rare, as light leads to oxygen production, and are limited to sulfidic outlets of the deep biosphere. For more on this see www.mpi-bremen.de/Judith-Klatt.page.
Although the modern mats no doubt differ from those that made the 3 gA old fossils, we can learn a lot about the old world from them.
A highly interesting site is the 'Little Salt Spring' in Florida. This oasis of Florida's recent past natural beauty is currently surrounded by retirement residences, hospitals and funeral homes. Hopefully it can resist the developers. It is a sinkhole, funnel shaped and stratified. Below 2 m depth is perfect anoxia, and at the lake floor at 9 m depth red microbial mats grow inhabited by Cyanobacteria. We found that in this perfect anoxic habitat, a few hours per day oxygen developed. This sliver of oxygen in the upper mm of the mats can sustain the presence of aerobic bacteria (sulfide oxidisers and nitrifiers). Probably, this minute and ephemeral oxygenation in the upper layer of mats occured long before the GOE, and critically, prepared life for age of oxygen. Both defenses against reactive oxygen (e.g. catalase) and respiration on oxygen were ready by small populations that developed in these tiny pockets.
de Beer, D., et al., Oxygenic and anoxygenic photosynthesis in a microbial mat from an anoxic and sulfidic spring. Environ. Microbiol., 2017. 19(3): p. 1251–1265
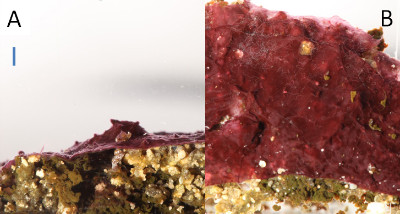
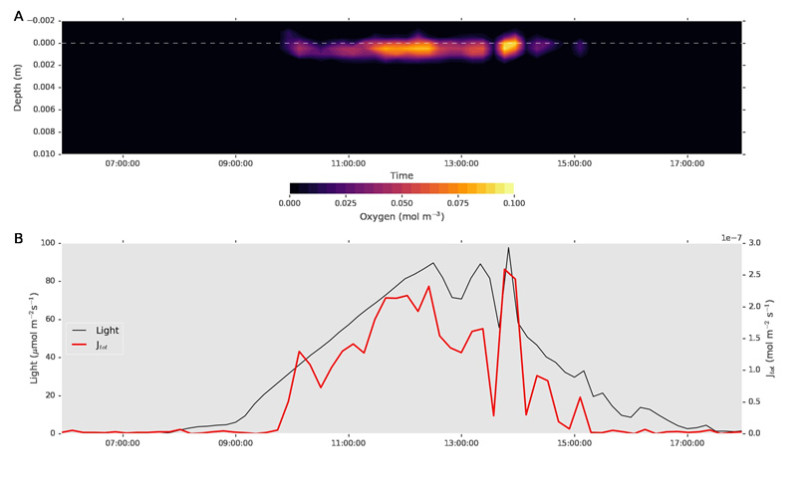
Sabkha
with Raeid Abed ([Bitte aktivieren Sie Javascript]), Andreas Greve ([Bitte aktivieren Sie Javascript]), Arjun Chennu ([Bitte aktivieren Sie Javascript]), Dimitri Meyer ([Bitte aktivieren Sie Javascript])
Sabkhas consist of salt-covered sediments, found in hot and dry climates. The salinity is saturated halite, yet they contain a diverse and dense microbiome. It was thought that the salt layer protects the deeper layers from dehydration and irradiation (Baas Becking, L.G.M., Geobiologie of inleiding tot de milieukunde. 1934, Den Haag: Van Stockum & Zoon; McKay, C.P., et al., PLoS ONE, 2016. 11(3): p. e0150342-e0150342).
This appeared not true: radiation and gasses pass the salt unhindered.
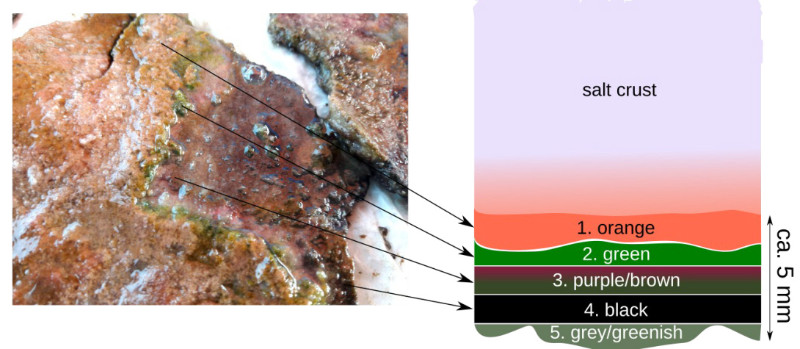
The microbes under the salt layer appeared not active. Only after inundation by seawater (as irregularly happens), high metabolic rates were observed (photosynthesis, respiration, sulfate reduction).
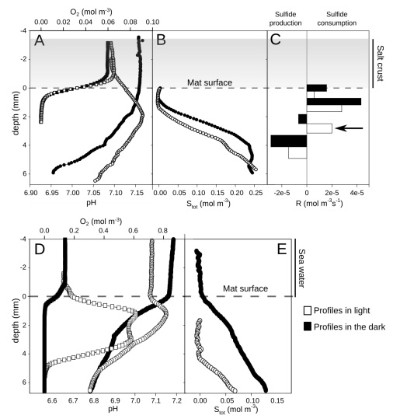
The two zones are observed in pigment distribution and light spectra, and molecular data show two different communities. The lower one is probably sulfide resistent, the upper one not.
Crucial is that the sabkha is hardly active during the dry period. No net primary production is possible, as anoxygenic phototrophy is coupled to sulfate reduction.
To sustain an active ecosystem periods of decreased salinity are needed.
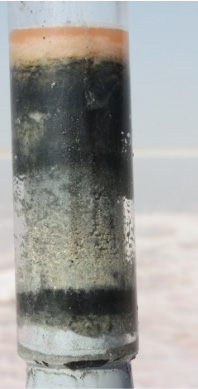
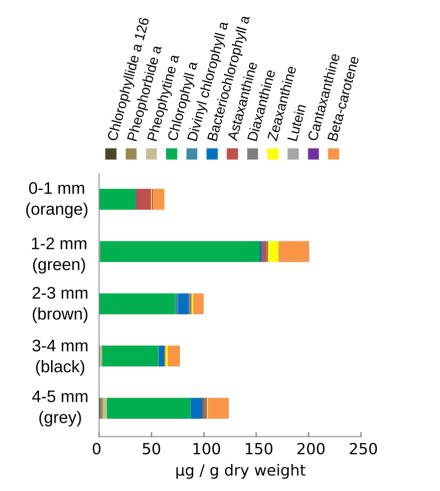